General Introduction
Antiferromagnetic Magnonics
Quantum spin-wave excitations (dubbed magnons) are charge neutral quasiparticles that can carry and transmit spin angular momenta without physical movements of electrons, hence eliminating Joule heating. Therefore, magnetic insulators can function as "metals" to conduct spins while blocking charges.
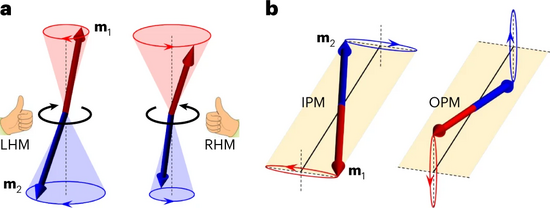
Adapted from Nature Materials 22, 684 (2023)
Being able to transport spins, however, does not automatically grant magnons a similar level of physical significance as electrons. In particular, the electron spin serves as an internal degree of freedom that can be manipulated via electromagnetic fields. By contrast, the magnon spin polarization in a ferromagnetic material is always opposite to the local magnetization, which is essentially fixed.
The situation is fundamentally different for antiferromagnetic materials (AFMs) in which neighboring magnetic moments compensate. In a collinear AFM with uniaxial anisotropy, symmetry guarantees that spin-up and spin-down magnons coexist, forming an intrinsic degree of freedom capable of encoding information. This unique feature opens an intriguing playground to explore the magnonic counterparts of pure spin phenomena usually associated with electrons. For example, the magnon spin Nernst effect refers to the generation of a pure spin current transverse to a temperature gradient, which can be regarded as the magnonic analog of the spin Hall effect. Another non-trivial example is that magnons in AFMs can function as electrons and mediate magnon-transfer torques (in a fully insulating spin valve).
The transport properties of magnons entail profound physical implications related to the geometric phase (a.k.a. Berry phase), which arises from the non-trivial phase relation of the spin waves. The hidden topology of the magnonic band structures reflected in the transport phenomena is often not perceptible in traditional perspectives.
- Quantum Behavior: Magnons are bosons that exhibit zero-point quantum fluctuations. At ultra-low temperatures, quantum effects become prominent as thermal magnons are suppressed. For example, the quantum energy of antiferromagnetic magnons confined by two adjacent magnets depends on the relative orientation of the two magnets, which leads to the magnon-mediated interlayer coupling (or, magnonic thermal Casimir effect) acting on the magnetization.
- Topological Magnons: Similar to electrons, the Berry phase associated with magnons gives rise to topologically protected edge states and chiral spin currents confined on the boundaries. In particular, when the magnon edge states appear near zero energy, they can be easily populated by thermal agitations (because magnons are bosons), leading to exotic thermo-magnetic behavior not available for electrons.
Antiferromagnetic Spintronics
AFMs can be operated in the ultrafast terahertz regime (1012 Hz, about 2 to 3 orders of magnitude faster than ferromagnets) by virtue of the "amplification" of the strong exchange interaction. In addition, AFMs have vanishing macroscopic magnetization so that they do not produce stray fields and are insensitive to magnetic perturbations.
Being one of the early pioneers in the emerging frontier of antiferromagnetic spintronics, we strive to unravel the fundamental mechanisms behind a wide variety of transport phenomena in antiferromagnetic materials.
The terahertz dynamics in AFMs can pump pure spin currents into an adjacent conductor via spin pumping across the interface, which enables the electronic detection (or sensing) of the terahertz dynamics. Reciprocally, a spin current flowing towards an AFM can generate terahertz spin dynamics through the spin-transfer torques. Theses effects manifest the interconversion of spin angular momenta among different physical systems that usually involve intricate spin-charge dynamics, forming one of the central inquiries in the emerging frontier of antiferromagnetic spintronics.
Concerning the spin interconversion, what is unique to AFMs is that the sublattice degree of freedom entangles with the spin. Consequently, electrons transferring into and out of an AFM will mingle spins with pseudo-spins. In addition, the coexistence of opposite spin species, which corresponds to the left- and right-handed chirality of spin precession in a classical picture, allows selective excitation of the spin polarization in the AFM-based spin pumping.
Monostructural Topological Spin-Insulatronics
A prevailing pursuit of spintronics is to effectively control magnetism through electrical stimuli, which is exemplified by a heterostructure composed of a magnet and a non-magnetic driver operated by electric currents. This archetypal setup, however, suffers from two fundamental problems:
-
The transfer of spin angular momenta is greatly inhibited by the interface
-
The electric power is mostly dissipated by Joule heating, rendering the overall efficiency surprisingly low.
The discovery of intrinsic magnetic topological insulators (iMTIs) opened a unique physical platform to address these two issues. Thanks to the intertwined topological electrons and magnetic ordering, an iMTI driven by electric fields can generate non-equilibrium spins on its own, which in turn drives its magnetic dynamics internally without the aid of other materials. Such a monostructural system can function as an electrical actuator and a magnetic oscillator simultaneously, obviating the undesirable interface in heterostructures. Furthermore, the spin generation and transfer processes do not rely on Ohm’s currents but are instead enabled by the “adiabatic currents” arising from the Berry curvature crosslinking the crystal momentum and magnetic orientation. Because adiabatic currents do not incur Joule heating, they can convert 100% of the input electric power into magnetic dynamics through the voltage-induced spin-orbit torque (SOT).
While the lossless SOT in iMTIs is enabled by the spin-orbit interaction, which correlates but is not determined by the band topology, there should be other possibilities where the lossless SOT arises from more exotic couplings among different degrees of freedom involving, for example, layer, orbitals, crystalline, and so on. With a broad vision inspired by (but not limited to) the iMTIs, this project makes inquiries into the following outstanding questions:
-
How to generically model and quantify the lossless SOT & adiabatic pumping?
-
What are the dynamical consequences of their combined effect?
-
Is the SOT mainly a bulk effect or an edge effect?
-
What is a proper topological description of the lossless SOT?
-
How to experimentally probe and characterize the lossless SOT?
Physics-Enabled Novel Applications
It is an appealing development in spintronics to recognize AFMs as promising candidate materials for next-generation device applications. AFMs can potentially lead to transformative technological advances because of the their salient physical features.
- Terahertz: Ultrafast terahertz operations of AFMs arise from unique physics rather than smart designs, holding huge potential to break the theoretical limit of ferromagnet-based electronics. "Bridging the terahertz gap" has been a century-long conundrum in science and engineering. AFM-based spintronics opens up an exciting opportunity.
- Spin: AFMs support magnons of opposite spin polarizations. This unique degree of freedom enables magnons to serve as active information carriers. Since magnons do not incur Joule heating, they can preserve and deliver spin information over long distances with very low dissipation. Moreover, it is theoretically possible to coherently manipulate the magnon spin and process information beyond binary, opening new possibilities for quantum computing.
- Robustness: Macroscopic magnetization vanishes in most AFMs, so AFM-based devices are free from stray fields, hence the crosstalk, between adjacent memory units. Exploiting AFMs as active spintronic materials can significantly improve the scalability and immunity of nanodevices.
- W.M. Keck Foundation (2024 - Present)
-
NSF CAREER award (2024 - Present)
-
DoD MURI award (2019 - Present)
-
Regents' Faculty Development award (Intramural, 2024 - Present)
-
Regents' Faculty Fellowships (Intramural, 2021 - 2022)
-
Omnibus Research and Travel award (Intramural, 2020 - 2021)
-
DoE-EFRC (SHINES center) Seed fund (Intramural, 2019 - 2020)
In PRL 132, 136701 (2024), we formulate and quantify the lossless SOT and its inverse effect, adiabatic charge pumping, in MnBi2Te4 of a few septuple-layer (SL) thick. The SOT is manifestly SL resolved and displays an evident even-vs-odd contrast of the (total) SL number. The physical consequences of the SOT are demonstrated by the SOT-induced magnetic resonances, where in the 3-SL case we identify a unique chiral mode that is blind to microwave electromagnetic fields but can be excited only by the SL-resolved SOT.
In contrast to conventional SOTs accompanied by charge currents, the SOT in MnBi2Te4 is not accompanied by Ohm’s conduction as the Fermi level lies in the gap under the charge-neutral condition. In our scenario, the output current only originates from the dynamics of magnetic moments as a reciprocal effect of the SOT, which is a pure adiabatic effect producing zero Joule heating. Under the combined action of the SOT and the adiabatic charge pumping, a voltage-driven MnBi2Te4 acquires an effective reactance (capacitive or inductive), whereby 100% of the input electric power can be converted into magnetic dynamics to overcome the Gilbert damping, achieving an unprecedented high efficiency of electrical manipulation of magnetism using a single material.
This work laid the theoretical basis for my NSF CAREER project and the collaborative project sponsored by the W.M. Keck foundation.
In magnetic topological insulators, quantized electronic transport is intertwined with spontaneous magnetic ordering, as magnetization controls band gaps, hence band topology, through the exchange interaction. In PRL 126, 026601 (2021), we show that considering the exchange gaps at the mean-field level is inadequate to predict phase transitions between electronic states of distinct topology. Thermal spin fluctuations disturbing the magnetization can act as frozen disorders that strongly scatter electrons, appreciably reducing the onset temperature of quantized transport even in the absence of structural impurities. This counterintuitive effect, which has hitherto been overlooked, provides an alternative explanation to several recent experiments on magnetic topological insulators.
The unique mechanism studied in this work is entirely different from the ordinary magnon-electron scattering. First of all, we have considered the adiabatic regime such that spin fluctuations are frozen in time, whereas magnons are propagating spin waves. Second, spin fluctuations form a background random potential that scatters the electrons passively, while reversely, the excitation of spin fluctuations by electrons is ignored. Third, the physical picture of spin fluctuations persists up to Tc, whereas magnons are well defined only at low temperatures. In addition, the mechanism here is intrinsic and independent of structure impurities.
The intrinsic magnetic topological insulator MnBi2Te4 is believed to be an axion insulator in its antiferromagnetic ground state. However, the direct identification of axion insulators remains experimentally elusive because the observed vanishing Hall resistance, while indicating the onset of the topological axion field, is inadequate to distinguish the system from a trivial normal insulator. In PRR 4, L022067 (2022) , we propose an axion-insulator magnetic tunnel junction (AMTJ) consisting of a few-layer MnBi2Te4 sandwiched between two metallic contacts as an experimental setup to unambiguously identify axion insulators through the quantized topological magnetoelectric effect. Using numerical Green's functions, we theoretically demonstrate the quantized magnetoelectric current in the proposed AMTJ, which can serve as a smoking-gun signal to unambiguously confirm axion insulators. Our predictions have inspired a series of experimental attempts.
For an sample of lateral size Lx = Ly = 10 µm, a harmonic magnetic field of strength B ~ 100 Gs and frequency ω/2π = 1 GHz induces an output ac current of amplitude 121.5 nA, which is a significant effect easy to detect.
Exotic physics in twisted bilayer 2D materials is a surging frontier in condensed matter physics. However, prevailing studies predominantly focus on the electronic structures associated with the moiré pattern while the hidden roles of phonons and magnons are largely overlooked. In PRB 102, 094404 (2020), we perform an in-time and in-depth investigation of the moiré magnons in twisted bilayer 2D magnets, assuming that the out-of-plane collinear magnetic order is preserved under weak interlayer coupling. By calculating the magnonic band structures and the topological Chern numbers for four representative cases, we find:
- The valley moiré bands are extremely flat over a wide range of continuous twist angles
- The topological Chern numbers of the lowest few flat bands vary significantly with the twist angle
- The lowest few topological flat bands in bilayer AFMs enable nontrivial thermal spin transport in the transverse direction
These properties make twisted bilayer magnets an ideal platform to study the magnonic counterparts of moiré electrons, where the statistical distinction between magnons and electrons plays a critical role.
Magnons in AFMs and their physical implications have helped facilitate the emergence of novel device concepts. A unique characteristic of antiferromagnetic magnons is the coexistence of opposite spin polarization, which mimics the electron spin in a variety of transport phenomena. Among them, the most prominent spin-contrasting phenomenon is the magnon spin Nernst effect (SNE), which refers to the generation of a transverse pure magnon spin current through a longitudinal temperature gradient. The magnon SNE can be intuitively regarded as the magnonic counterpart of the spin-Hall effect. In APL 120, 090502 (2022), we introduce selected recent progress in the study of magnon SNE with focus on its underlying physical mechanisms. In a related study published in PR Applied 16, 034035 (2021), we critically examined the physical challenges obstructing the electronic detection of the magnon SNE, suggesting that optical measurements should be more reliable to experimentally verify this effect.
It is well established that the spin-orbit interaction in heavy metal/ferromagnet heterostructures leads to a significant interfacial Dzyaloshinskii-Moriya interaction (DMI), which modifies the internal structure of magnetic domain walls (DWs) to favor Néel- over Bloch-type configurations. However, the impact of such a transition on the structure and stability of internal DW defects (e.g., vertical Bloch lines) awaits exploration. In PRB 99, 184412 (2019), we present an integrated analytical-micromagnetic study to describe a type of topological excitation called DW skyrmion characterized by a 360° rotation of the internal magnetization in a Dzyaloshinskii DW. We further propose a method to identify DW skyrmions experimentally using Fresnel-mode Lorentz transmission electron microscopy; simulated images of DW skyrmions using this technique are presented based on the micromagnetic results.
Although open questions remain about their thermal stability and dynamic properties, DW skyrmions provide an alternative strategy for leveraging topological protection in magnetic systems with a strong interfacial DMI. The reconfigurable nature of the DWs that host these excitations could open the door to different kinds of memory and computing schemes based on topological charge.
Collaborating groups led by: Vincent Sokalski & Marc De Graef (CMU), Claudia Mewes & Tim Mewes (Univ Alabama)
Ferrimagnets (FIMs) can function as high-frequency AFMs while being easy to detect as ferromagnets, offering unique opportunities for ultrafast device applications. While the physical behavior of FIMs near the compensation point has been widely studied, there lacks a generic understanding of FIMs where the ratio of sublattice spins can vary freely between the ferromagnetic and antiferromagnetic limits. In PRB 105, 064410 (2022), we investigate the physical properties of a two-sublattice FIM manipulated by static magnetic fields and current-induced torques. By continuously varying the ratio of sublattice spins, we clarify how the dynamical chiral modes in a FIM are intrinsically connected to their ferro- and antiferromagnetic counterparts, which reveals unique features not visible near the compensation point. In particular, we find that current-induced torques can trigger spontaneous oscillation of the terahertz exchange mode. Compared with its realization in AFMs, a spin-torque oscillator using FIMs not only has a reduced threshold current density but also can be self-stabilized, obviating the need for dynamic feedback.
Spin pumping converting sub-terahertz electromagnetic waves to DC spin currents has recently been demonstrated in AFMs with easy-axis magnetic anisotropy. However, easy-plane AFMs such as NiO, which are easier to prepare experimentally, are considered to be bad candidates for spin pumping because the Néel vector oscillation is linearly polarized, placing a major restriction on the material choice for practical applications. Through a case study of NiO in APL 121, 072401 (2022) , we show that an applied magnetic field below the spin–flop transition can substantially modify the polarization of the resonance eigenmodes, which enables coherent sub-terahertz spin pumping as strong as that in easy-axis AFMs. In addition, we find that an applied magnetic field can significantly reduce the threshold of Néel vector auto-oscillation triggered by spin-transfer torques. These prominent field-assisted effects can greatly facilitate spintronic device engineering in the sub-terahertz frequency regime.
This work was partially inspired by the experimental realization of spin pumping in a canted easy-plane AFM (details can be found below), and as a matter of fact, challenged the seemingly established picture that "spin pumping does not work for easy-plane AFMs" we previously argued in PRL 2014. Indeed, scientific progress is always built on overturning previous conclusions.
Through two independent experiments using different materials (MnF2: Science 2020 and Cr2O3: Nature 2020), which corroborate each other, my theoretical prediction of the terahertz spin pumping in AFM had been verified experimentally, marking a major breakthrough in the field of antiferromagnetic spintronics.

Collaborating groups led by:
Enrique Del Barco (UCF), David Lederman (UCSC),
Johan van Tol (FSU), Arne Brataas (NTNU)
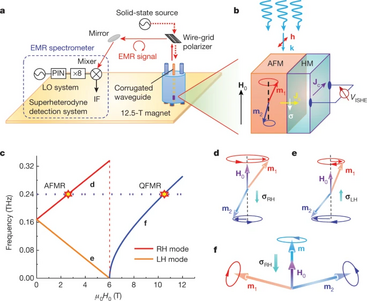
Collaborating groups led by:
Jing Shi (UCR), Mark Sherwin (UCSB), Peng Wei (UCR)
The realization of sub-terahertz spin pumping in AFMs inspired a flurry of experiments worldwide, including a collaboration between our group and the former Nanoscale Quantum Sensing group at UCSD. In PRL 127, 117202 (2021), we report dc spin pumping by the acoustic resonant mode in a canted easy-plane antiferromagnet 𝛼−Fe2O3 enabled by the Dzyaloshinskii–Moriya interaction. Systematic angle and frequency-dependent measurements demonstrate that the observed spin-pumping signals arise from resonance-induced spin injection and inverse spin Hall effect in 𝛼−Fe2O3—metal heterostructures, mimicking the behavior of spin pumping in conventional ferromagnet-nonmagnet systems. The pure spin current nature is further corroborated by reversal of the polarity of spin-pumping signals when the spin detector is switched from platinum to tungsten which has an opposite sign of the spin Hall angle. Our results reveal the intriguing physics underlying the low-frequency spin dynamics and transport in canted easy-plane antiferromagnet-based heterostructures.
Collaborating groups led by: Chunhui Du (UCSD)
SOT-driven deterministic control of the magnetic state of a ferromagnet with perpendicular magnetic anisotropy is key to next-generation spintronic applications including non-volatile, ultrafast and energy-efficient data-storage devices. However, field-free deterministic switching of perpendicular magnetization remains a challenge because it requires an out-of-plane antidamping torque, which is not allowed in conventional spin-source materials such as heavy metals and topological insulators due to the system’s symmetry. The exploitation of low-crystal symmetries in emergent quantum materials offers a unique approach to achieve SOTs with unconventional forms. In Nat. Mater. 2022, we report field-free deterministic magnetic switching of a perpendicularly polarized van der Waals magnet employing an out-of-plane antidamping SOT generated in layered WTe2, a quantum material with a low-symmetry crystal structure. Our numerical simulations suggest that the out-of-plane antidamping torque in WTe2 is essential to explain the observed magnetization switching.
Major collaborating groups led by: Simranjeet Singh & Jyoti Katoch (CMU)
The effect of spin currents on the magnetic order of insulating AFMs is of fundamental interest and can enable new applications. Toward this goal, characterizing the SOTs associated with AFM–heavy metal interfaces is important. In PRL 2022, we report the full angular dependence of the harmonic Hall voltages in a predominantly easy-plane AFM, epitaxial c-axis oriented 𝛼−Fe2O3 films, with an interface to Pt. By modeling the harmonic Hall signals together with the 𝛼−Fe2O3 magnetic parameters, we determine the amplitudes of field-like and damping-like SOTs. Out-of-plane field scans are shown to be essential to determining the damping-like component of the torques. In contrast to ferromagnetic–heavy-metal heterostructures, our results demonstrate that the field-like torques are significantly larger than the damping-like torques, which we correlate with the presence of a large imaginary component of the interface spin-mixing conductance.
Our work demonstrates a direct way of characterizing SOTs in AFM-heavy metal heterostructures, opening up a promising path for future studies on similar AFM-HM heterostructures as well as a means that can be used in optimizing SOTs on AFM for applications.
Collaborating groups led by: Andrew Kent (NYU), Fengyuan Yang (OSU)
Unidirectional spin Hall magnetoresistance (USMR) has been widely reported in the heavy metal/ferromagnet bilayer systems. In PRL 2023, we report the observation of the USMR in Pt/𝛼−Fe2O3 bilayers where the 𝛼−Fe2O3 is an antiferromagnetic insulator. Systematic field and temperature dependent measurements confirm the magnonic origin of the USMR. The appearance of USMR is driven by the imbalance of creation and annihilation of AFM magnons by the SOT due to the thermal random field. However, unlike its ferromagnetic counterpart, theoretical modeling reveals that the USMR in Pt/𝛼−Fe2O3 is determined by the antiferromagnetic magnon number with a non-monotonic field dependence. Our findings extend the generality of the USMR which pave the ways for the highly sensitive detection of AFM spin state.
Collaborating groups led by: Kang Wang (UCLA), Fengyuan Yang (OSU)
Terahertz spin dynamics and vanishing stray field make AFMs the most promising candidate for the next-generation magnetic memory technology with revolutionary storage density and writing speed. However, owing to the extremely large exchange energy barriers, energy-efficient manipulation has been a fundamental challenge in AFMs. In Nat. Comm. 2022, we report an electrical writing of antiferromagnetic orders through a record-low current density on the order of 106 A/cm facilitated by the unique AFM-FM phase transition in FeRh. By introducing a transient FM state via current-induced Joule heating, the SOT can switch the AFM order parameter by 90° with a reduced writing current density similar to ordinary FM materials. This mechanism is further verified by measuring the temperature and magnetic bias field dependences, where the X-ray magnetic linear dichroism (XMLD) results confirm the AFM switching besides the electrical transport measurement. Our findings demonstrate the exciting possibility of writing operations in AFM-based devices with a lower current density, opening a new pathway towards pure AFM memory applications.
Major collaborating groups led by: Kang Wang (UCLA)
It has long been speculated that multiferroic materials could bring unique physical properties, hence new functionalities, to electronic devices. For example, it is far from clear whether (and how) the multiferroicity could lead to unique control of spin transmission mediated by magnon excitations. In established approaches, magnons are mostly manipulated through magnetic dipoles (that is, by breaking time-reversal symmetry). In Nat. Mater. 2024, we report a highly non-trivial experimental observation of chiral spin transport in multiferroic BiFeO3 and its control by reversing the ferroelectric polarization (that is, by breaking spatial inversion symmetry). The ferroelectrically controlled magnons show up to 18% modulation at room temperature. The spin torque that the magnons in BiFeO3 carry can be used to efficiently switch the magnetization of adjacent magnets, with a spin–torque efficiency comparable to the spin Hall effect in heavy metals. Utilizing such controllable magnon generation and transmission in BiFeO3, an all-oxide, energy-scalable logic is demonstrated composed of spin–orbit injection, detection and magnetoelectric control. Our observations open a new chapter of multiferroic magnons and pave another path towards low-dissipation nanoelectronics.
Major collaborating groups led by: Ramamoorthy Ramesh & Zhi Yao (UC Berkeley), Daniel Ralph (Cornell)
Magnetic topological materials with coexisting magnetism and nontrivial band structures exhibit many novel quantum phenomena, including the quantum anomalous Hall effect, the axion insulator state, and the Weyl semimetal phase. As a stoichiometric layered antiferromagnetic topological insulator, thin films of MnBi2Te4 show fascinating even–odd layer-dependent physics. In Nano Letters 2024, we report the observation of the Chern insulator state at high magnetic fields in a series of thin-flake MnBi2Te4 devices fabricated using stencil masks. Upon magnetic field training, a large exchange bias effect is observed in odd but not in even septuple layer (SL) devices. Through theoretical calculations, we attribute the even–odd layer-dependent exchange bias effect to the contrasting surface and bulk magnetic properties of MnBi2Te4 devices. Our findings reveal the microscopic magnetic configuration of MnBi2Te4 thin flakes and highlight the challenges in replicating the zero magnetic field quantum anomalous Hall effect in odd SL MnBi2Te4 devices.
Major collaborating groups led by: Cui-zu Chang (PSU)